WHAT!??, you say. Excuse me, but it does not matter how clean it is, it is still a GHG emitter, you say. What about the mountains be killed in West Virginia, you say.
No, not mineral coal. Not the fossil fuel.
Charcoal.
I came across this post on The Oil Drum by engineer poet, of Ergosphere, and halfway down into a very long post ... it had morphed into a charcoal diary.
Well, by gawd, this diary is going to be a charcoal diary from the get go. Springboarding from, as engineer poet's did, the "flashcarb" work on high efficiency charcoal production with electricity co-generation done by the Hawaii Natural Energy Institute (HNIE).
And, don't you know, it ties right back into Great Lakes Windpower, to boot. So "flashcarb", coppice and standards, and complementing Great Lakes windpower, after the fold.
So, where does this fit in?
Now, I don't believe in "silver bullets". Rather, I believe in hunting for a variety of different silver shotgun pellets, and packing as many as possible into our Sustainable Energy Independence policy initiatives. So the point of describing some of the HNIE research below is that this is one promising approach to converting biomass into a useful form for energy production.
And what makes it especially promising is the way that it complements Windpower. For example, consider the following figure taken from the manfrommiddletown contribution to the Booman Tribune blog on Great Lakes Windpower. It plots the wind power at two locations in Michian, with the stronger wind resource on the coast:
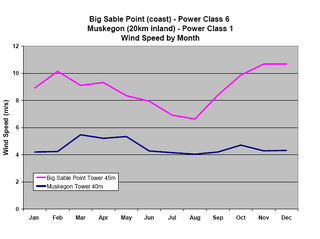
So, what to do about that? One of the most efficient ways of storing up Windpower in surplus periods for later use is pumped-storage hydroelectric, which is already in use at, for example, the Luddington Pumped Storage Plant in Western Michigan:
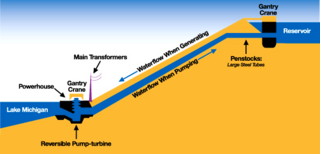
Indeed, one of the appeals of Lake Erie for Windpower is the availability of coastal locations that are 100 meters above water level, which allows for efficient pumped-storage hydropower. That is combined with an average depth of 19 metres, where present commercial offshore Windpower projects are limited to 20 metres depth (although I would be surprised if deeper water projects are not developed in the decades ahead).
However, It makes for lower capital cost if we are storing Windpower for the coming week, rather than for six months in the future.
And this is what makes charcoal from Flash Carbonization a promising complement to Great Lakes Windpower. Part of energy that is recovered in this process is best used to make electricity immediately. And the charcoal itself is readily stored, so we can be flexible about the precise timing of the charcoal production.
The fundamental strategy here is therefore to increase the rate of charcoal production during periods of peak electricity demand. This increases the financial return on the process, because the excess electricity generated during the process can be sold into the grid at a premium price.
And once the charcoal has been produced, the charcoal itself can be used to bring additional baseline electricity production online during the summer months.
Where Does the Biomass come from?
HNIE have performed experiments on charcoal production with a variety of feedstocks, including wood sources such as Oak and Leucaena, and agricultural wastes such as Corn Stover, including Corn Cobs.
The charcoal typically contains more than half the energy of the original biomass, but less than a third of the weight, so it is an weight-saving process. The process of charcoal production itself does not have extreme scale economies, so it makes a certain amount of economic and energy sense to produce the charcoal near to the biomass source.
Some of the biomass would be agricultural wastes, which could be converted by farmer co-ops into charcoal and electricity.
The other main source of biomass would be wood plantations. And that brings us back to the last period of intensive technological development of charcoal, before we launched into this extended detour with mineral coal. This is the coppice system of wood production for charcoal.
What is coppice? Coppice is harvesting a tree for perennial production by cutting it down near to the roots, allowing the base and root system to remain in place so that new shoots can emerge in the following year. Coppice is harvested in a rotation, on a cycle that varies from one type of tree to the next.
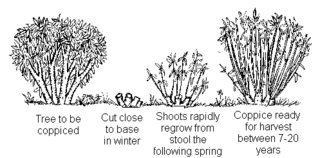
And, of course, if we harvest coppice from hill or mountain along the contours of the terrain, the retention of the tree roots systems combines with the natural terracing effect of contour farming to retain both soil and rainfall runoff far better than clearfell timbering.
Henry the VIII introduced regulations that required a certain number of large trees, "standards", to grow in the midst of the coppice woodlot. This was, of course, a "strategic defense" reserve, at the time, since the Royal Navy needed timber to make their Wooden Ships.
Today, the most pressing strategic issue we face is avoiding the destruction of the ecosystem services that we rely upon for our survival. And so we probably should steal a trick from the House of Tudor, and incorporate a similar system in a contour-coppicing system. This would involve rewarding producers for allocating, say, 10% of land to ridgeline and mountaintop forest reserves, and 10% of contours to production of larger timbers.
What About the Politics of the System?
As an economist and an amateur policy wonk, I am most excited by the natural "fit" in this system. Long experience informs us, however, that any system will run aground if the politics conspire against it. And when a system runs aground on the shoals of political infighting, an elegant "fit" just makes the loss that much more poignant.
In this case, though, I reckon that the politics could just possibly work out.
Many of the areas where Big Coal has the most sway are areas that could reasonably aspire to become biomass producers for charcoal production. And unlike mineral coal production, this is not just production of the primary product. This would also includes production of peak load electricity at locations distributed across Southeastern Ohio, West Virginia, and Eastern Kentucky.
And when this is combined with additional cash incomes to the farmers of rural Ohio for their agricultural wastes, it means that almost every part of the state gains a direct stake in some part of this system.
Any new electricity generation capacity brought online to use the charcoal ought to be a cleaner process such as a direct Carbon Conversion Fuel Cell. However, from where we stand today, charcoal offers utility companies an opportunity to start weaning themselves off of mineral coal, and therefore provides an opportunity for driving a political wedge between Big Coal and their current allies among electricity suppliers.
Flash Carbonization
Of course, the devil is in the details, and most of the details that I have on hand are from papers published in technical journals. That means that they are likely to be accessible to people with ties to a University (and in particular with a Research University), but access by just regular folks costs money. So while I will give the citation references below, I will delve just a little bit into some of the details here.
The process that HNIE called "flashcarb" is based on producing a batch of charcoal under pressure. An electric coil ignites the feedstock at the bottom. A flash fire is formed that moves from the bottom of the chamber to the top, and air is fed in from the top of the chamber at the same pressure, expelling the exhaust gas from the bottom and captured for further processing.
The key to the conversion efficiency of the process is the exhaust gas, which is a hot mixture of various volatile gases, including hydrogen and methane. For example, with one version of the set-up, an experiment with a feedstock of Leucaena (a nitrogen-fixing tree) resulting in more than 59% of the feedstock carbon fixed in the charcoal, and 65% of the moist mass of the Leucaena gasified. And in those experiments, air-dried Leucaena performed as well as debarked oven-dried Leucaena, which would allow those energy-using steps to be skipped (2003: 3695).
Indeed, to quote from the 2003 paper giving research results at that time:
- When moist (ca. 13%) Leucaena wood is carbonized, the product gas has a HHV of 3000 kJ/kg of dry Leucaena feed or 17% of the energy content of the Leucaena. Copious amounts of steam (e.g., 0.49 kg/kg of Leucaena) also are generated at elevated pressure (e.g., 1 MPa) during the flash carbonization of moist feedstocks that can be delivered to a turbine to generate shaft power. (2003: 3698)
Why isn't the carbon burning up? It would appear to be one of those sciency kind of things ...
... Because of the high combustion rate of the secondary vapors in the presence of oxygen at elevated pressure, and mass-transfer limitations that prevent the oxygen from reaching the surface of the carbon, flash carbonization is a remarkably quick and efficient method for converting biomass into carbon. (2003: 3698)
But, isn't charcoal production a dirty process?
Well, yes, certainly, if two guys in the African rain forest sneak out one day, cut down a tree, build a charcoal pit, and use that to make charcoal, which they bring to market ... yeah, that's a dirty operation.
This, however, is a whole other kettle of fish. Since the process takes place under pressure, the container is already air-tight, so it is straighforward to channel the exhaust for further processing. And it is the second stage combustion of the exhaust gas that is the key. This relies on a catalyctic afterburner which eliminates the carbon monoxide which is present in the unprocessed exhaust gas. When the exhaust gas was combined with air to maintain an oxygen level of about 4%:
the concentrations of carbon monoxide in the effluent were, in almost all cases, lower than the detection limit of the GC analysis performed in this study ([less than] 300 ppm). At the same time, methane was also almost completely combusted in the afterburner. (2006: 595)
Citations
Michael Jerry Antal, Jr., Kazuhiro Mochidzuki, and Lloyd S. Paredes. (2003) "Flash Carbonization of Biomass." Industrial & Engineering Chemistry Research Vol. 42, pp. 3690-9.
Teppei Nunoura, Samuel R. Wade, Jared P. Bourke, and Michael Jerry Antal, Jr. (2006) "Studies of the Flash Carbonization Process. 1. Propagation of the Flaming Pyrolysis Reaction and Performance of a Catalytic Afterburner." Industrial & Engineering Chemistry Research Vol. 45, pp. 585-99.